Bioengineered model of human intestine could be used for drug testing and personalised medicine
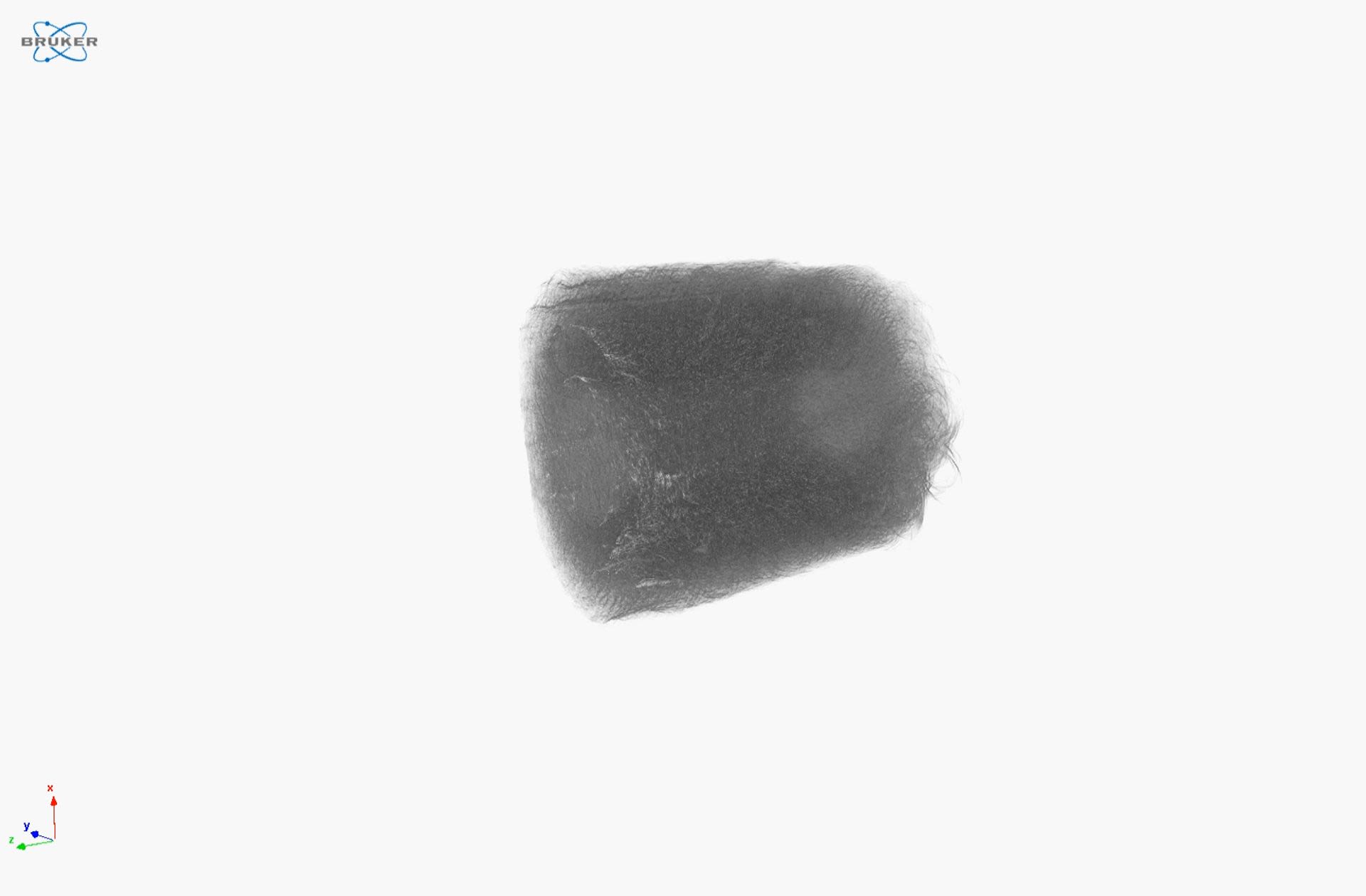
Researchers from our Bioelectronic Systems Technology (BEST) Group have developed a 3D, bioengineered model of the human intestine that uses a smart material scaffold to enable continuous in-line monitoring of the cells and tissues.
Their design enables them to grow different intestinal cells together, in a 3D structure that mimics that of an in vivo human intestine. The group hope the model can be used to test potential drug candidates, with a view to replacing, or at least complementing and reducing, some animal testing models, as well as enabling the study of diseases that occur in the gut.
“The gut is a tissue with a lot of interest behind it,” says Chrysanthi-Maria Moysidou, co-lead author on the paper and final year PhD student in the BEST group. “If we talk about drug testing and drug screening applications, particularly for drugs that we take orally, intestinal models are very important because it is the epithelial barrier of the gut that the drug substances will have to cross before they reach our bloodstream and through that their target tissue/organ in our body.”
There is huge demand for in vitro models and in vitro human tissues for the study of disease mechanisms and for drug or treatment development, as they can provide results that are more relevant to the human system than animal models. The field of organs-on-chips is a growing technology in this area, using scaffolds and supports combined with microfluidics to grow miniaturised human tissues, with some of these chips now being commercialised.
Most currently available models are two-dimensional – cells growing in a layer across a flat surface – and therefore don’t accurately mimic the shape, structure, and function of these tissues in the human body. The methods used to validate results from these models also require disruption of the system: components need to be chemically labelled and probed, while samples need to be sectioned for optical analysis with microscopy.
“As impressive and as useful as these models are, they lack in-line monitoring components,” explains Moysidou. “Some of the assays would be destructive or would rely on downstream analysis only. But for models that run for longer term, in studies that take weeks or months, how are you going to be sure that everything is running smoothly?”.
This is where the BEST group’s smart material comes in. They use an electrically active, biocompatible organic polymer called PEDOT:PSS as the substrate on which to grow the cells. Using this polymer, they have designed a special tube-shaped scaffold to mimic the three-dimensional shape of the human intestine.
Different cells give off different electrical signals, which can provide information on cell health, growth and activity. Because the scaffold on which the cells grow is conducting, the researchers can continuously monitor these signals to track how their tissue is developing.
“This in-line, real-time monitoring helps us make prompt decisions about the tissue status, the health of the tissue, if the cells differentiate or not for example. Particularly for intestinal cells which form a barrier tissue, we monitor the resistance of this barrier, which is an important property that reflects how tight and intact the barrier is and hence how effectively it regulates transport of ions, solutes and other molecules.”
Photograph of the well‐plate assembly, incorporating the tubular device constructs. The devices are mounted in a six well‐plate using a push‐fit connection (seen in black), keeping the electrical terminals outside each well (white wires).
Photograph of the well‐plate assembly, incorporating the tubular device constructs. The devices are mounted in a six well‐plate using a push‐fit connection (seen in black), keeping the electrical terminals outside each well (white wires).
SEM images showing the transverse (left) and the longitudinal (right) section of the luminal PEDOT:PSS scaffolds.
SEM images showing the transverse (left) and the longitudinal (right) section of the luminal PEDOT:PSS scaffolds.
To visualise the 3D structure of their PEDOT:PSS scaffold, Moysidou worked with Mohammed Al-Sharabi, a PhD student in our Terahertz Applications Group, to use a technique called X-ray microcomputed tomography (XμCT).
A video showing how X-ray microcomputed tomography can visualise the PEDOT:PSS tube in 3D and at different cross-sections throughout the sample
A video showing how X-ray microcomputed tomography can visualise the PEDOT:PSS tube in 3D and at different cross-sections throughout the sample
“The XμCT technique characterises the microstructure properties of materials in a non-destructive manner, so the sample remains intact during the measurement,” says Al-Sharabi. “We can visualise the microstructure of the sample at any position of interest throughout the sample without having to physically slice or cut the sample and XμCT can be used to visualise the sample in 3D, as well as calculate its porosity.”
“That was super important for us because otherwise we wouldn’t be able to be that accurate in calculating the porosity,” explains Moysidou. “This property is key for building our bioelectronic model because it helps us understand what the available space is for cells to infiltrate the scaffold and it helps us evaluate various things about cell coverage and cell seeding and in turn design the strategy for building tissues.”
There are two key cell types that make up the intestine in this 3D gut model: epithelial cells, which in the native tissue act as the barrier between what we ingest and our bloodstream, and a type of connective tissue layer, here formed by a type of cells known as a fibroblasts. This connective tissue layer was hosted in the spongy bulk compartment of the tubular conducting scaffolds, with the layer of epithelial cells sitting on top of it, lining the central channel, or ‘lumen’, of the tube.
“To make sure we get an in vivo-like tissue organisation, we had to come up with this two-stage seeding strategy,” explains Moysidou. “So we said let’s leave the fibroblasts inside the scaffold for a few days, let them grow, cover all the pores, and then let’s inject the intestinal cells in the lumen lining, where they are supposed to be, and see if the fibroblasts will act as a guide to make sure the intestinal cells stay where they need to stay. And as we saw with optical analysis of the models at the end of the experiment, this approach to guide cells to auto-organise as they would in vivo, worked.”
The model is a key component of the group’s wider project, ‘IMBIBE’, in which they are aiming to build a multi-organ platform that can mimic communication between the gut, the brain and also the microbiome.
“The intestinal microbes play a critical role for our health but they also seem to be involved in many disease phenotypes,” says Moysidou. “We can see that a person who suffers from depression or has Parkinson’s, for example, has a different microbiome from a healthy individual, but we don’t know if this change is a symptom or a cause of the disease.
“The microbiota also play a critical role in metabolising the drugs we might take for any disease. There is evidence for Parkinson’s that a commonly used drug for this condition is metabolised by specific microbes in our gut so that the amount of drug that reaches the brain is much less than we would expect, and this is why that drug is not as effective for some patients. Because each of us has a community of microbes in our guts as unique as our fingerprints, we also need models to study all of these effects and use them in personalised medicine prognostic and diagnostic applications.”
They hope the model will be useful for drug testing, allowing studies on human cells and tissue that may be more relevant than traditional models and animal studies. “This manuscript represents a tremendous amount of work culminating in the delivery of an advanced 3D model of the human intestine,” says Professor Róisín Owens, who leads the BEST group. “We look forward to seeing how our model can be used to advance medical research.”
Upper: A schematic illustration of the bioelectronic platform hosting the human intestine model and magnified x/z orthogonal views (ortho‐views, right) of the samples obtained by z‐stacked confocal images. Lower: Immunostained transverse, longitudinal, and orthogonal sections of the 3D bioelectronic intestinal model, demonstrating enteric cells forming a continuous epithelial layer on the lumen lining supported by the lamina propria layer in the bulk compartment of the L‐Tubistor scaffolds.
Upper: A schematic illustration of the bioelectronic platform hosting the human intestine model and magnified x/z orthogonal views (ortho‐views, right) of the samples obtained by z‐stacked confocal images. Lower: Immunostained transverse, longitudinal, and orthogonal sections of the 3D bioelectronic intestinal model, demonstrating enteric cells forming a continuous epithelial layer on the lumen lining supported by the lamina propria layer in the bulk compartment of the L‐Tubistor scaffolds.
Read the full paper, published in Advanced Biology:
Moysidou, C.‐M., Pitsalidis, C., Al‐Sharabi, M., Withers, A. M., Zeitler, J. A., Owens, R. M., 3D Bioelectronic Model of the Human Intestine. Adv. Biology 2021, 2000306. https://doi.org/10.1002/adbi.202000306
This work is part of the European Research Council supported project, IMBIBE, Grant Number: 723951. Mohammed Al-Sharabi’s research is sponsored by Johnson Matthey and EPSRC.