Sculpting DNA to Build the Future of Synthetic Cells
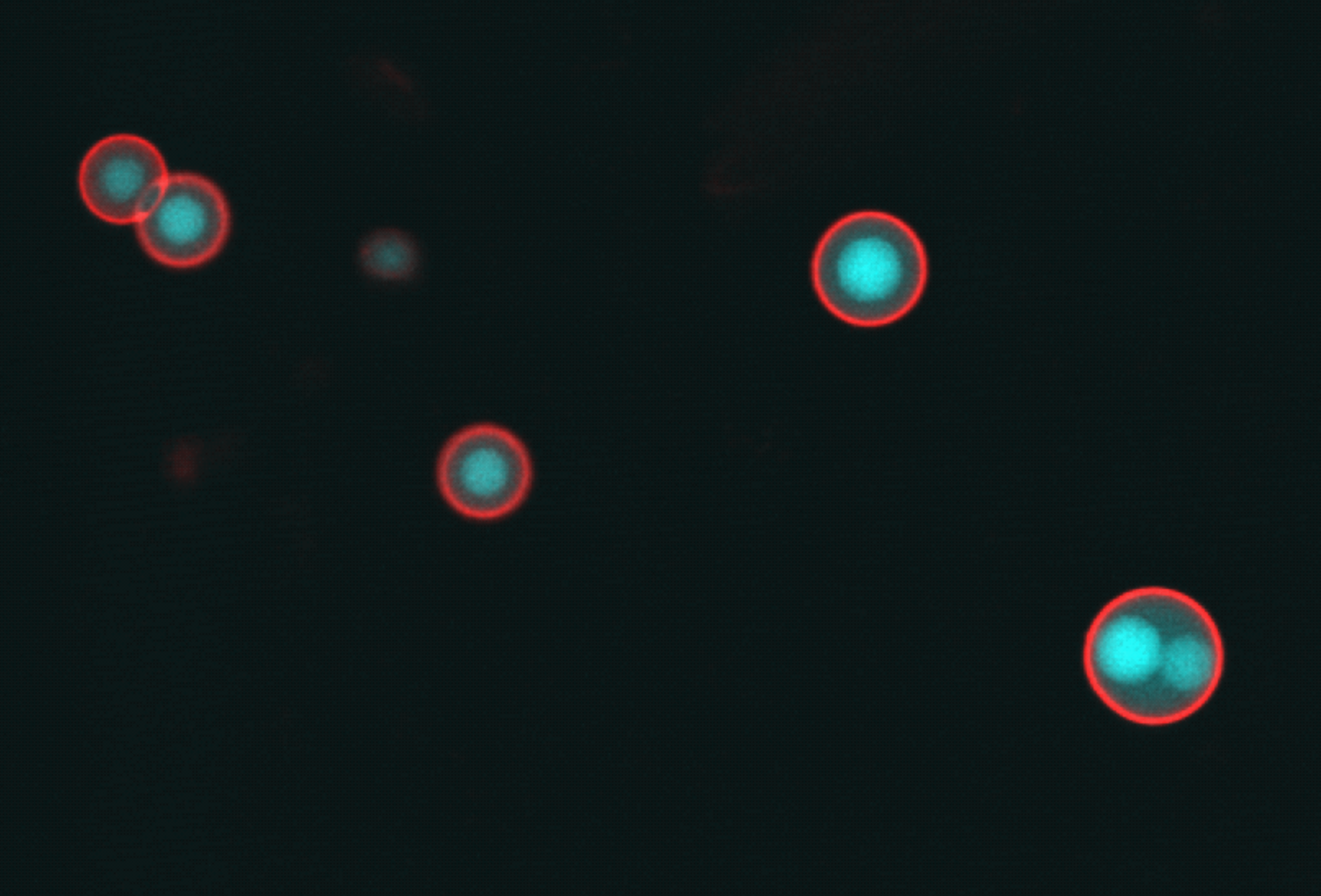
Researchers from the Department of Chemical Engineering and Biotechnology (CEB) have made a significant advancement in the area of synthetic biology, unveiling a novel method to design synthetic cells using DNA building blocks. This pioneering work promises to reshape our understanding and capabilities within the field.
Led by Dr Lorenzo Di Michele, first author Layla Malouf and the research team designed unique DNA nanostructures that self-assemble into complex sub-compartments and respond to specific inputs. These structures resemble living cells in both appearance and functionality, containing multiple spatially organised organelles which can host reactions similar to some of those found in living cells.
The Cambridge researchers have tapped into the inherently programmable ability of DNA to self-assemble, thereby creating cell-like scaffolds that offer a more straightforward and efficient avenue to synthetic cell development. The approach developed by the team overcomes many of the challenges associated with more traditional methods of making synthetic cell scaffolds. Layla Malouf remarks:
“By combining our DNA nanostructures with other modular building blocks, we’ve shown how a simple process can be used to generate complex structures without the need for expensive equipment or time-consuming synthesis and purification steps,”
The team’s use of amphiphilic DNA nanostructures drives the self-assembly of large structures that display an internal complexity similar to what is seen in natural cells. Among the impressive features of their research is the creation of a "pseudo-membrane", structures analogous to a cell's cytoplasm, membrane-less organelles, and the capacity to support RNA synthesis. Such feats highlight the possibility that systems, even with a limited set of molecular building blocks, can reflect the intricate structural and functional dynamics of living cells.
Diving deeper into the methodology, the synthetic cells are crafted to have multiple capabilities: the first, to fall apart when triggered by a specific input, and the second to house a specific DNA blueprint. When activated, this blueprint leads to the production of RNA. Interestingly, this production process also causes a temporary "swelling" of the synthetic cells, mirroring morphological changes seen in biological cells.
Dr. Lorenzo Di Michele expressed enthusiasm about the research, stating:
"Our methodology showcases the incredible potential that DNA nanotechnology holds. With just a handful of distinct building blocks, we've managed to emulate some of the architectural and functional richness seen in biological cells. The range of potential applications is vast, from medicine to environmental solutions and much more."
Another exciting outcome of the research is the identification of key design rules for making complex, DNA-based, cell-sized structures. With these rules to guide them, other researchers can now use this approach to construct functional synthetic cells for their own work.
These synthetic cells could serve multiple purposes. They have the ability to store and gradually release specific genetic materials, which could usher in ground-breaking medical treatments, including innovative vaccines or gene therapies. Furthermore, the cells can accommodate other functional elements, such as enzymes or nanoparticles. The outer shell, primarily lipid-based, also presents numerous possibilities. It can be tailored to hold onto specific molecules, merge with other cells, or serve a myriad of other specialised tasks.
Concluding the insights from their research, the team believes their approach holds the key to designing multi-functional synthetic cells. Given the right design, these cells could tackle many roles, from the production of genetic material to targeted drug delivery. It's evident that with DNA leading the charge, the horizon of synthetic biology appears more promising than ever before.
The full paper can be accessed here
Driven by curiosity. Driving change