Energy Reactions and Carriers
Ewa Marek
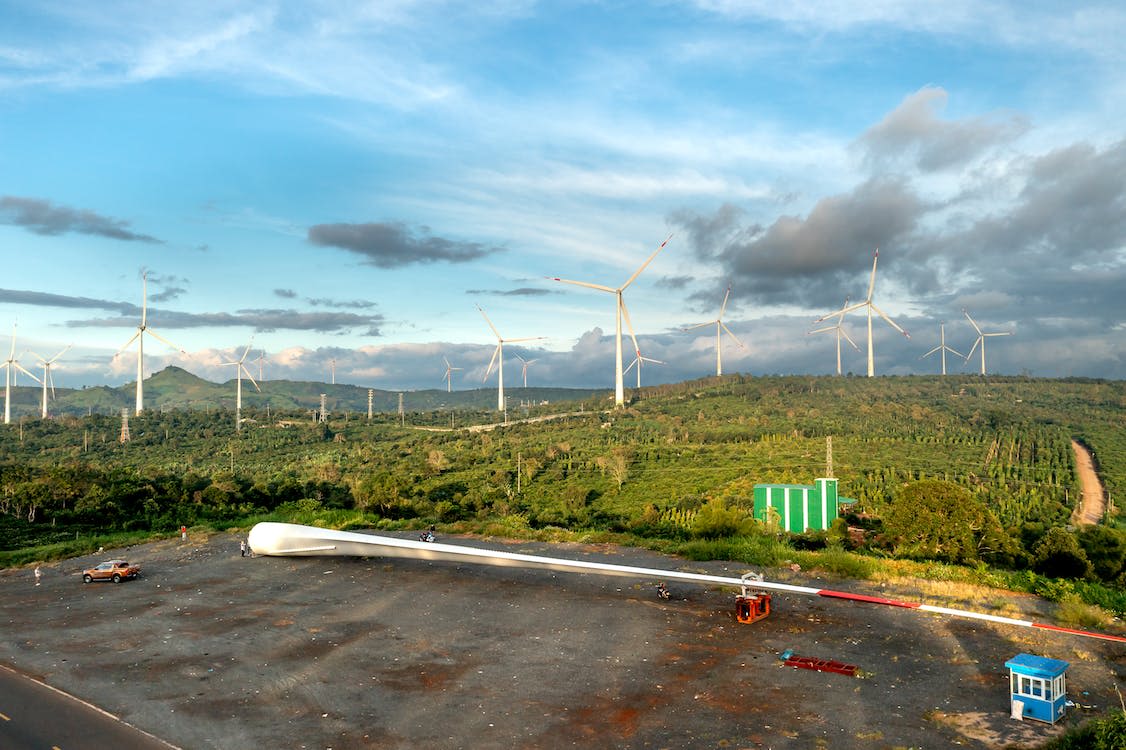
We undertake research in negative and net-zero energy technology, thermochemical energy storage, sustainable production of high-value chemicals for Net-Zero Chemical Industry, physics of granular flow, sustainable processes and processing. We also investigate the production, application and processing of new fuels and energy vectors (ammonia, hydrogen, synthetic hydrocarbons, and solid carriers).
About us
Group Leader
Previous Group Leaders
Assistant Professor for Teaching
Post Doctorate Research Associate
Undergraduate Students
Alexandra Anastasiadou
Tsoi Chow
Jamie Dosdale
Mathush Kantharupan
Hari Prasad
Jake Stokes
Previous Students
Bryan Ooi
Luigi Rizzo
Salar Namin
Zoltan Terjek
Swiss Research Foundation Fellow
Dr Xiaoyu Dai
Postgraduate students
Raymond Chend
Cameron Few
Aki Fujinawa
George Fulham
Joe Gebers
Abu Kasim
Paula Mendoza Moreno
Yujia Tracy Wang
Visiting researchers
Dr Magda Olak-Kucharczyk
Dr Natalia Festinger Lukasiewicz
Research
New processes utilizing biomass or waste (garbage, industrial waste) for electricity production with efficient capture of CO2.
The focus is primarily on fluidised bed reactors, since they are important in novel cycles using solid fuels for power generation, designed to capture and sequester the CO2. Fluidised combustors are very important in power generation using biomass fuels, since they give advantages over other combustors in (i) tolerance to changes in fuel, (ii) the catalysis of reactions or capture of pollutants by the bed material, (iii) high heat transfer, and (iv) economic operation at relatively small scales. A particular interest is Chemical Looping (CL). In basic CL, gaseous fuel is oxidised by a solid metal oxide (or oxygen carrier), MeO, in one reactor. The exit gas yields almost pure CO2 after the steam is condensed. The reduced metal oxide, Me, is transferred to an oxidation reactor and regenerated. Thus, the fuel is combusted, but the CO2 is separated from the nitrogen in air. CL with solid fuels is more difficult because fuel and carrier are not easy to separate during carrier regeneration. Ensuring durability of the MeO over many redox cycles is an important problem being studied by the Group. We also work on the use of iron and other oxides to produce clean hydrogen from biomass, uncontaminated with COx and suitable for fuel cells. Multi-institutional collaborations, elucidating the materials-science of looping-materials, are used to relate process performance to structure.
Gasifying solid fuels to CO and H2 allows the enhanced watergas-shift (WGS) process, using solid CaO to remove the carbon content as CaCO3, to produce hydrogen for fuel. Subsequent heating of CaCO3 releases the CO2 for sequestration. The absorption capacity of CaO from natural minerals falls markedly with number of forward and reverse steps, but we have discovered ways of reducing this fall. The Group has also created synthetic sorbents which decay in capacity much less than natural sorbents. Other research has examined (i) the kinetics of fuel devolatilisation, (ii) theory and experiment on mass transfer around combusting, or gasifying particles in fluidised beds of oxygen carrier, (iii) the reaction between SO2 and calcium-based sorbents and (iv) attrition and break-up of oxygen carriers in fluidised beds.
Chemical looping for gas-phase, selective oxidation reactions.
In a new departure for the CL area, we are examining (in collaboration with Johnson Matthey plc) whether structured particles, containing mixtures of catalyst and oxygen donor, can be used to effect selective oxidation reactions, e.g. the industrially-important reactions of oxidative dehydrogenation of alkanes to alkenes and the epoxidation of ethylene and propylene. The critical point is that the organic does not have to be mixed with low levels of air – the oxygen is provided in lattice form from the MeO. The significance is that success would enable these reactions to be run more safely, more intensively and with greater selectivity than is currently possible. Cambridge Enterprise has invested in a patent application for epoxidation (Chan et al. 2017) and proof of principle is currently being demonstrated with the target being to exceed selectivity and conversion seen in industrial reactors using the conventional process.
Physics of granular flows.
Processes in (1) depend on efficient gas-solid reactions and solids flow. A primary interest is fluidisation, investigated using innovative combinations of new (e.g. Magnetic Resonance Imaging, MRI) and existing (e.g. Particle Image Velocimetry, Electrical Capacitance Tomography) experimental techniques, underpinned by theoretical approaches (e.g. Discrete Element Modelling, DEM).Granular systems are visually opaque, making observations difficult inside three-dimensional (3D) systems. Accordingly, most previous experimental studies have been restricted to so-called two-dimensional (2D) fluidised beds, but the fluid dynamics are influenced by the walls and the findings cannot be confidently generalised to 3D beds. Until recently, only two experimental techniques have existed for the investigation of opaque, two-phase granular systems in 3D; X-radiography and electrical capacitance tomography (ECT), both of which present significant problems. In collaboration with Prof. Gladden, we have developed magnetic resonance (MR) imaging to observe fluidised beds internally. The significant advantage of MR over other techniques is that it can image the distribution of solids as well as their velocities and can image the gas. Research has shown the unique capability of ultra-fast (viz. acquisition times of 1-25 ms) MR measurements to give detailed, quantitative information on complex phenomena occurring in 3D gas-fluidised beds. As a result, a number of areas of long-standing controversy have finally been settled. For example, on whether gas issues from the holes in the distributor as a stream of bubbles or as a permanent jet. This is important, practically, because the distributor region can markedly affect overall performance in industrial units. For scale-up, we have conducted the first comparison of MRI with Electrical Capacitance Tomography, ECT (with Prof. L-S. Fan, Ohio State) on fast-fluidisation. We have also cross-validated MRI with X-ray imaging (Prof. P. Lettieri, UCL) and also with PEPT (Dr D. Parker, Birmingham U.). For fluidised beds, vibrating beds and other granular flow reactors, Discrete Element Modelling (DEM) has been used and involves coupling the equations of motion of each individual particle with the equations describing the fluid mechanics of the gas and solving the whole simultaneously for incremental steps in time, allowing for the mechanics of deformation of the particles as they collide. The purpose is to understand, e.g. the movement of reacting fuel particles, which is only possible with a realistic description of both gas and solid phases present. A very important facet is to validate the theoretical predictions with experimental results from MR and PIV, an aspect neglected by other modellers because of the inaccessibility of 3D systems to direct observation. One recent PhD student (see papers by Boyce et al., below) has used special MR techniques to track both gas and solids simultaneously – a major achievement.
Sustainable processes and processing.
We have undertaken reaction engineering studies of the Fischer-Tropsch (FT) synthesis and methanation using CO and CO2. The research is largely concerned with understanding the fundamental interactions between mass transfer and intrinsic chemical kinetics, particularly as liquid are formed in FT.
In a recent project, we have been examining solar-driven water splitting, focusing on Au/TiO2 systems, which have been previously reported to be active under visible light. The aim is to produce hydrogen. We have examined the efficiency of water splitting on disordered titanate nanotubes and titania surfaces activated with gold nanoparticles; of interest here is developing an understanding of how the combination of properties of the gold nanoparticles and nanotubes will affect the efficiency of water splitting under visible light. We have also experimented with structured nanotube systems and special perovskite materials and investigated how their properties can be altered by the structure and modification with other components. Our target is to construct proof of principle devices to demonstrate the feasibility of using both the disordered and ordered systems for photocatalytic water splitting.
The group has undertaken system-level, life-cycle studies of, e.g. proposed routes for converting biomass to fuel or products. A recent PhD project studied the opportunities for making the production of polyethylene terephthalate (PET, used in making bottles for drinks and in fibres) more sustainable by sourcing one or more of the raw materials from crop-derived materials.
Other research is focusing on the evaluation at both pilot scale and bench scale of continuous ion exchange chromatography to remove particular products from a process stream derived from a fermentation. Based on this, the collaborating company will be able to decide whether or not the technology will be suitable for an envisaged, large-scale process. Further research in this separations area has examined a novel solvent-extraction approach for isolating butanol, resulting in a patentable discovery (Hodgson & Dennis 2018).
We publish regularly in these fields, including attendance at major international conferences, and have significant links with researchers around the world.